Original research | Peer reviewed |
Cite as: Pittman JS, Myers GH, Stalder KJ, et al. Effect of fenbendazole on shedding and embryonation of Ascaris suum eggs from naturally infected sows. J Swine Health Prod. 2015;23(5):252–263.
Also available as a PDF.
SummaryObjectives: To determine reduction of Ascaris suum egg shedding and ovicidal effects in naturally infected commercial female breeding swine treated with fenbendazole. Materials and methods: Five shedding and three embryonation experiments across three commercial sow farms were conducted. Ascaris suum-infected sows were allocated to four treatments: untreated controls; 545.5 mg fenbendazole, 1 day (Treatment 1); 545.5 mg fenbendazole, 3 consecutive days (1636.5 mg total) (Treatment 2); and 1636.5 mg fenbendazole, 1 day (Treatment 3). Fecal samples were collected and evaluated by a standard flotation method (shedding study) or eggs were isolated and incubated (embryonation study) to determine embryonation rates. Groups were compared for time-to-negative (Kaplan-Meier survival analysis); percent negative (chi-square analysis); environmental burden (analysis of variance); and embryonation rates (analysis of variance). Results: Time-to-negative ranges were 9.3-13.1, 8.9-13.1, and 9.8 days post treatment (DPT) for treatments 1, 2, and 3, respectively; control ranges were 13.4-28.2 DPT. Treatment sows were 90%-100% negative, compared to 0.0%-28.6% of controls. Environmental burden ranges were 7.0%-60.9%, 13.9%-60.8%, and 29.3% (treatments 1, 2, and 3, respectively) and 60.4%-219.0% for controls. All treatment values differed from controls (P < .05), but not from each other. Embryonation rates were lower for treatments than controls at 6 and 8 DPT (P < .001). Implications: Fenbendazole at various dosages is effective against A suum infections in sows. Treatment should begin 14 days prior to movement into clean farrowing facilities. Under the conditions of this study, fenbendazole demonstrates ovicidal activity against A suum at 4-8 DPT. | ResumenObjetivos: Determinar la reducción de la excreción de huevos Ascaris suum y de los efectos ovicidas en hembras comerciales infectadas tratadas con fenbendazol. Materiales y métodos: Se realizaron cinco experimentos de excreción y tres de formación de embrión en tres granjas comerciales de hembras. Las hembras infectadas con el Ascaris suum fueron asignadas a cuatro tratamientos: control sin tratamiento; 545.5 mg de fenbendazol por 1 día (Tratamiento 1); 545.5 mg de fenbendazol por 3 días consecutivos (1636.5 mg en total) (Tratamiento 2); y 1636.5 mg de fenbendazol por 1 día (Tratamiento 3). Se recolectaron muestras fecales y se evaluaron por medio del método de flotación estándar (estudio de excreción) o se aislaron los huevos y se incubaron (estudio de formación de embrión) para determinar los índices de formación de embrión. Se compararon los grupos de tiempo a negativo (análisis de supervivencia Kaplan-Meier); porcentaje negativo (análisis de chi-cuadrado); carga medioambiental (análisis de varianza); e índices de formación de embrión (análisis de varianza). Resultados: Los rangos de tiempo a negativo fueron 9.3-13.1, 8.9-13.1, y 9.8 días post tratamiento (DPT por sus siglas en inglés) para los tratamientos 1, 2, y 3, respectivamente; los índices de control fueron 13.4-28.2 DPT. Las hembras en tratamiento fueron 90%-100% negativas, comparadas con un 0.0%-28.6% del grupo control. Los índices de carga medioambiental fueron 7.0%-60.9%, 13.9%-60.8%, y 29.3% para los tratamientos 1, 2, y 3, respectivamente, y 60.4%-219.0% para control. Todos los valores de los tratamientos difirieron del control (P < .05), pero no entre ellos. Los índices de formación de embrión fueron más bajos en los tratamientos que en control a 6 y 8 DPT (P < .001). Implicaciones: El fenbendazol en diferentes dosis es efectivo contra las infecciones de A suum en hembras. El tratamiento debe comenzar 14 días antes del cambio a instalaciones de maternidad limpias. Bajo las condiciones de este estudio, el fenbendazol demuestra actividad ovicida contra el A suum entre 4-8 DPT. | ResuméObjectifs: Déterminer la réduction d’excrétion d’œufs d’Ascaris suum et les effets ovicides chez des truies d’élevages commerciaux naturellement infectées traitées avec du fenbendazole. Matériels et méthodes: Cinq essais en lien l’excrétion et trois avec l’embryonnation ont été réalisés dans trois fermes commerciales de reproduction. Des truies infectées par A suum ont été réparties dans quatre groupes de traitement: témoin non-traité; 545,5 mg de fenbendazole pour 1 journée (Traitement 1); 545,5 mg de fenbendazole pour 3 jours consécutifs (1636,5 mg au total) (Traitement 2); et 1636,5 mg de fenbendazole pour 1 journée (Traitement 3). Des échantillons de fèces furent prélevés et évalués par la méthode standard de flottaison (étude sur l’excrétion) ou les œufs furent isolés et incubés (étude sur l’embryonnation) afin de déterminer les taux d’embryonnation. Les groupes ont été comparés quant au délai pour devenir négatif (analyse de survie Kaplan-Meier); le pourcentage d’animaux négatifs (chi-carré); la charge environnementale (analyse de variance); et taux d’embryonnation (analyse de variance). Résultats: L’écart des délais pour devenir négatif étaient de 9,3-13,1; 8,9-13,1; et 9,8 jours post-traitement (JPT) pour les traitements 1, 2, et 3, respectivement; l’écart pour le groupe témoin était de 13,4-28,2 JPT. Les taux de négativité variaient entre 90%-100% pour les truies traitées, comparativement à 0%-28,6% pour les témoins. Les charges environnementales variaient entre 7,0%-60,9%; 13,9%-60,8%; et 29,3% pour les traitements 1, 2, et 3 respectivement, et 60,4%-219,0% pour les témoins. Les valeurs des groupes traitées différaient toutes significativement (P < 0,05) de celles du groupe témoin mais pas entre elles. Les taux d’embryonnation étaient moindres à 6 et 8 JPT pour les groupes traités comparativement aux témoins (P < 0,001). Implications: L’administration de fenbendazole à différents dosages est efficace contre une infection par A suum chez les truies. Le traitement devrait débuter 14 jours avant le déplacement dans des installations de mise-bas propres. Dans les conditions expérimentales de cette étude, le fenbendazole a démontré une activité ovicide contre A suum à 4-8 JPT. |
Keywords: swine, fenbendazole, Ascaris suum, roundworm, sows
Search the AASV web site
for pages with similar keywords.
Received: July 2, 2014
Accepted: December 12, 2014
Ascaris suum, the large roundworm of swine, is the most common and important parasite of swine, with worldwide distribution.1,2 The main economic impacts of A suum on swine are reduced feed efficiency,3,4 decreased average daily gain,5-7 liver condemnations at slaughter due to larval migration and organ pathology (“milk spots”),8-10 and increased medication costs related to treatment.11,12
Additional A suum impacts include suboptimal vaccine efficacy associated with migrating larvae;13,14 organ damage from larval migration, predisposing the host to secondary diseases;15-24 morbidity and mortality associated with acute infection;25-27 and impacts on carcass and meat quality.28,29 Ascaris suum may be a zoonotic infection in areas where humans and swine cohabitate.30-33
Significant changes in the way most modern swine are managed, such as housing pigs indoors, separation of ages and production phases, improved sanitation, and effective anthelmintic use, have either eliminated or significantly reduced parasite incidence and severity. Due to reduced prevalence and overdispersion within populations,34 parasites cause mainly a subclinical disease and not often considered to be of major importance in modern swine production.12,35 However, due to several A suum characteristics, the parasite still persists in modern production systems.1,36-39 Those characteristics are a highly fecund adult female (estimated to produce 1 to 2 million eggs per day for up to 55 weeks),40,41 eggs that are highly resistant to environmental conditions and disinfectants,42-44 a direct life cycle involving extra-intestinal migration,45 and a relatively short pre-patent period.45 The high environmental contamination level and egg resistance make it nearly impossible to eradicate A suum from contaminated facilities, thus continuous monitoring and implementation of control measures are required.35,44
Recent husbandry requirements driven by concerns other than parasite control, such as group sow housing, required provision of nesting or bedding materials, and drug use restrictions, are re-introducing known risk factors and creating exposures that promote A suum transmission, resulting in increased prevalence and clinical severity within those farm types.46-49 In addition, niche production systems (eg, organic, pasture-raised, differentiated markets) and swine associated with regional and national exhibition show circuits provide environments for the parasite’s maintenance.50-54
The goal for most parasite control programs is to minimize clinical disease and production impact through preventing transmission and reducing environmental contamination.35,55-58 This is accomplished by improved sanitation, management of pig flow, and use of anthelmintics at key times in the parasite’s life cycle and host’s production cycle. On breeding farms, sanitizing the farrowing facilities before loading sows and removal of organic material on the skin of sows by washing prior to farrowing are often implemented as control measures.44,55-57 Another common practice in the swine industry is to administer anthelmintics to pregnant sows prior to entering clean farrowing facilities.35,44 This reduces transmission of eggs to the farrowing environment (eg, stall, pasture) and from the sow to piglets. Treatment timing prior to movement to farrowing may vary considerably, and if the period between treatment and moving into farrowing area is too short, egg shedding into the farrowing environment is not prevented.
Fenbendazole is a broad-spectrum benzimidazole (Class I) anthelmintic approved for use in swine in North America and elsewhere around the world. Fenbendazole has a high safety margin59,60 and is highly effective against the adult and larval stages of A suum.60-65 The benzimidazole class of anthelmintics has ovicidal activity against parasites of a number of species.66-70 This activity results from the benzimidazole molecule binding with embryonic tubulin at the leading edge of polymerization, which prevents microtubule formation.68,69,71
Fenbendazole is currently available in North America as a feed additive (Safe-Guard Medicated Dewormer for Swine; Merck Animal Health, Summit, New Jersey) or as an individual feed top-dress (Safe-Guard EZ Scoop; Merck Animal Health). The product is available for other species in North America and for swine in the other countries under the trade name Panacur (MSD Animal Health, Summit, New Jersey). The individual top-dress formulation enables treatment of individual animals or subpopulations (ie, weekly batches) without necessity of simultaneously medicating the entire population served by the feed system, and eliminates the need for on-farm feed mixing, which may not be available.
The use of fenbendazole in swine has been studied extensively, and its use in sow herds is common.72 However, little information is available regarding fenbendazole’s impact on shedding dynamics and embryonation of A suum eggs post treatment under common commercial conditions. Judicious drug use would be supported by evidence of the magnitude of impact on shedding, shedding duration, and time to negative shedding post treatment under commercial conditions. Understanding the dynamics of reduced environmental contamination is of benefit in developing effective control measures for A suum in commercial swine operations worldwide. Further knowledge on controlling A suum in swine may be used to model Ascaris lumbricoides control in humans.31,73
The series of experiments presented herein were conducted to characterize the impact of fenbendazole (Safe-Guard EZ Scoop) on A suum egg shedding and embryonation rates for A suum eggs that were shed from naturally infected gestating sows under commercial conditions that included commonly used anthelmintic protocols.
Materials and methods
All animals were cared for in accordance with the Guide for the Care and Use of Agricultural Animals in Research and Teaching (http://www.fass.org/docs/agguide3rd/Ag_Guide_3rd_ed.pdf) and Pork Quality Assurance Plus (PQA Plus) guidelines (http://www.pork.org/Certification/2341/pqaPlusMaterials.aspx).
Farms
The farms in which all experiments (five shedding and three embryonation experiments) were conducted were sow farms in a commercial swine production company, using Large White-Landrace cross maternal genotypes, between 2010 and 2013. Farms A, B, and C were selected because they had each been previously diagnosed with A suum infection in breeding sows as part of a system surveillance study reported by Pittman et al.37 Farm A was a 1000-sow, one-site, breed-to-market operation located in southeastern Virginia. Farms B and C were each 2000 breed-to-feeder-pig operations (feeder pigs 10 weeks of age), both located in northeastern North Carolina. All farms were managed with weekly batches of gestating, multiparous sows in which sows were housed in individual gestation and farrowing stalls, weaning pigs at approximately 3 weeks of age. Replacement gilts, purchased from an off-site multiplication flow, were housed in groups of four to six until bred, when they were moved into individual gestation stalls. All farms utilized fenbendazole (Safe-Guard EZ scoop) as a feed top-dress weekly for gestating sow groups 2 weeks prior to farrowing. All routine fenbendazole use was suspended prior to starting and for the duration of the experiments. Animals were fed individually through automated drop boxes once daily, and individual water nipples were available for each animal at all times.
Experiments
Egg shedding. Five separate shedding experiments were conducted among the three farms between February 2011 and December 2013: one each at Farm A and Farm B and three at Farm C. Each experiment differed in number of subjects, sample collection dates, and treatments, as detailed in Table 1. All subject enrollment, sample collection, sample processing, and application of treatments were consistent among shedding experiments. No animals were used in more than one shedding experiment.
Table 1: Five shedding experiments comparing fenbendazole treatment dosages and treatment periods for Ascaris suum in naturally infected gestating sows*
Experiment | Farm | Treatment | n | Fecal sample collection days | No. negative (%) | Time-to-negative (days) | Mean BURD (%) | ||
---|---|---|---|---|---|---|---|---|---|
Mean | SE | Range | |||||||
1 | A | CNT | 4 | 0, 4, 5, 6, 8, 10, 12, 14, 16, 18, 20 | 1 (25.0)a | 18.0a | NA | 18-20 | 136.6a |
TX1 | 7 | 7 (100.0)b | 13.1b | 0.9 | 10-16 | 46.4b | |||
TX2 | 10 | 10 (100.0)b | 11.0b | 1.2 | 4-18 | 36.1b | |||
2 | B | CNT | 5 | 0, 8, 10, 14 | 0 (0.0)a | 14.0a | NA | 14 | 79.9a |
TX1 | 6 | 6 (100.0)b | 9.3b | 1.0 | 8-14 | 60.9b | |||
TX2 | 10 | 9 (90.0)b | 10.6b | 0.8 | 8-14 | 60.8b | |||
3 | C | CNT | 10 | 0, 8, 10, 12, 14, 21, 24, 31 | 3 (30.0)a | 22.3a | 1.6 | 10-24 | 219.0a |
TX1 | 13 | 13 (100.0)b | 11.7b | 0.4 | 10-14 | 44.4b | |||
TX2 | 12 | 11 (91.7)b | 11.3b | 0.5 | 8-31 | 33.1b | |||
4 | C | CNT | 14 | 0, 4, 8, 10, 14, 22, 30, 37 | 3 (21.4)a | 28.2a | 1.5 | 14-37 | 60.4a |
TX1 | 29 | 28 (96.6)b | 11.5b | 0.7 | 4-22 | 7.0b | |||
TX2 | 26 | 26 (100.0)b | 13.1b | 1.0 | 4-22 | 13.9b | |||
5 | C | CNT | 7 | 0, 4, 6, 8, 10, 14, 21 | 2 (28.6)a | 13.4a | 0.8 | 10-21 | 118.6a |
TX1 | 12 | 12 (100.0)b | 9.5b | 0.6 | 6-14 | 32.4b | |||
TX2 | 9 | 9 (100.0)b | 8.9b | 0.8 | 6-14 | 20.6b | |||
TX3 | 11 | 11 (100.0)b | 9.8b | 0.7 | 8-14 | 29.3b |
* In five studies across three commercial sow farms, gestating sows (F1 Large White × Landrace, multiparous; n = 185) positive for A suum were each randomly allocated to one of four treatment groups: untreated controls (CNT); 545.5 mg fenbendazole (Safe-Guard EZ Scoop; Merck Animal Health, Summit, New Jersey) given on a single day (TX1); 545.5 mg fenbendazole given on each of 3 consecutive days (1636.5 mg fenbendazole total) (TX2); and 1636.5 mg fenbendazole given on a single day (TX3). Fecal samples were collected at various time points within each experiment for evaluation by fecal flotation and egg quantification. Treatment groups were compared by number negative at end of study period; time-to-negative (time to stop shedding) by survival analysis; and environmental burden (BURD), a measure of total egg shedding during the study.
a,b Values with different superscripts within a category and within an experiment are statistically significant (P < .05): number negative, chi-square with Fisher’s exact test; mean time-to-negative, Kaplan-Meier log-rank test; mean environmental burden, analysis of variance.
n = number of sows by experiment-treatment; SE = standard error; NA = not applicable.
Embryonation. Three separate embryonation experiments were conducted between January 2012 and December 2013. Each experiment differed in number of subjects, sample collection dates, and treatments, as detailed in Table 2. All subject enrollment, treatment applications, sample collection, sample processing, and embryonation evaluation were consistent among experiments. No animals were used in more than one embryonation experiment.
Table 2: Three embryonation experiments on a sow farm comparing three fenbendazole treatment dosages and treatment periods for Ascaris suum in naturally infected gestating sows*
Experiment | DPT | Treatment group | P | |||||||
---|---|---|---|---|---|---|---|---|---|---|
CNT | TX1 | TX2 | TX3 | |||||||
n | ER (SD) | n | ER (SD) | n | ER (SD) | n | ER (SD) | |||
1 | 8 | 11 | 95.4a (8.6) | 18 | 29.3b (24.2) | 10 | 30.5b (18.2) | ND | ND | < .001 |
2 | 0 | 13 | 92.8a (13.3) | 25 | 92.5a (17.4) | 21 | 85.0a (17.9) | ND | ND | NS |
8 | 11 | 95.6a (8.6) | 18 | 29.4b (24.0) | 11 | 38.2b (21.2) | ND | ND | < .001 | |
3 | 0 | 7 | 98.6a (2.9) | 12 | 97.8a (2.9) | 9 | 94.0a (5.6) | 11 | 97.1a (4.2) | NS |
2 | 7 | 99.1a (1.1) | 10 | 99.0a (1.5) | 9 | 93.1a (10.9) | 11 | 95.9a (8.7) | NS | |
4 | 7 | 99.3a (0.8) | 12 | 75.4a (31.0) | 8 | 70.9a (20.1) | 8 | 47.0b (34.1) | < .01 | |
6 | 7 | 90.3a (21.3) | 12 | 48.6b (20.9) | 8 | 28.6b (26.1) | 11 | 26.6b (28.9) | < .001 |
1 In three studies on a commercial sow farm, gestating sows (n = 137; described in Table 1) positive for A suum were each randomly allocated to one of four treatment groups (described in Table 1). Fecal samples were collected at various time points by experiment, and A suum eggs were isolated and incubated to determine embryonation rates. Treatment groups were compared by mean embryonation rates as a percent of ova reaching full larval development.
a,b Values within a row with different superscripts are statistically different (Tukey’s studentized range test).
DPT = days post treatment; ER = embryonation rate (percent); SD = standard deviation; ND = not done; NS = not significant (P > .05).
Sow inclusion and exclusion criteria. Inclusion and exclusion criteria were consistent for all experiments. On the basis of reported literature and previous observations in the herd, younger animals (gilts through second-parity sows) were initially screened for A suum infection status, because of a higher expected prevalence of infection.74-76 The goal was to identify gestating adult females shedding A suum eggs in their feces. In order to screen a large number of animals in an efficient and rapid manner, a modified fecal flotation method was utilized. Approximately 1 gram of feces was collected directly from the rectum of selected gilts and sows. A new clean nitrile glove was used for each animal to prevent sample cross-contamination. Each fecal sample was placed in a 15-mL centrifuge tube, pre-filled with 5 mL of a concentrated sugar solution (Sheather’s solution).77 Samples were processed immediately on site. Fecal samples and sugar solution were manually homogenized within the 15-mL tube by vigorous shaking. Supplemental homogenization, if required, was accomplished using a disposable wooden stirrer, discarded after a single use. A volume of sugar solution was added to each tube sufficient to create a reverse meniscus at the top of the tube. A 22 × 22-mm glass coverslip was placed on top of each tube and allowed to sit for 10 minutes minimum. Coverslips were removed, placed on glass microscope slides, and examined under 40× magnification for A suum eggs. Each coverslip was examined until confirmation of at least one egg was observed or no ova were visualized on the entire coverslip, ie, the sample was determined to be negative. In all experiments, animals providing positive test samples were enrolled in the study and randomly allocated using a random number generator to treatment and control groups, while animals with negative test samples were excluded from enrollment. For all enrolled animals, a day 0 fecal sample was collected and eggs per gram were quantified using the modified Wisconsin sugar flotation technique.78 Animals with a negative day 0 fecal test were considered to have been “false positives” on initial screening and were excluded from the remainder of the experiment.
Treatments. Treatments were applied in a manner consistent with existing on-farm processes and were consistent for all experiments. Safe-Guard EZ Scoop was used for all fenbendazole treatments, and the scoop provided in the package was used for subject dose allotments. The scoop when level full provides approximately 545.5 mg of fenbendazole, sufficient to provide 3 mg per kg bodyweight to a 181.8-kg animal. In common field application, a level full scoop is provided to each sow, regardless of bodyweight, and treatments were based on this methodology. Therefore, a single scoop was estimated to provide 545.5 mg fenbendazole for each animal.
Treatment groups for all experiments were as follows: non-treated control; a single 545.5-mg scoop of fenbendazole for 1 day (Treatment 1); a single 545.5-mg scoop of fenbendazole daily for 3 consecutive days, total 1636.5 mg (Treatment 2); or three 545.5-mg scoops of fenbendazole on a single day, total 1636.5 mg (Treatment 3).
Treatment was applied to sows’ individual feed drop boxes the day prior to feed being dispensed the following morning. Anthelmintic intake by each sow was monitored as complete intake of feed prior to the next feeding, and all animals in the studies consumed their entire daily ration.
Sample collection and processing
Egg shedding experiments. At each sample day, approximately 5 grams of feces was collected per rectum of each enrolled sow. If a fecal collection from a sow failed after two attempts, that sow was excluded from the day’s collection. After collection, fecal samples were placed into 50-mL screw-top centrifuge tubes and held at 4°C until processing within 24 to 72 hours after collection. Samples were processed using the modified Wisconsin sugar flotation method.78 Slides were evaluated using 40× magnification, and the number of A suum eggs on the entire slide was counted. A maximum egg count per slide (“cutoff”) of 500 eggs was utilized.
Embryonation experiments. Samples in Experiment 1 were collected on day 8 after the start of treatment. Samples in Experiment 2 were collected prior to treatment (day 0) and on day 8 after the start of treatment. Samples in Experiment 3 were collected on day 0, and on days 2, 4, and 6 after the start of treatment.
On each collection day, feces (approximately100 to 500 g) was collected per rectum in a clean plastic sample bag in a manner consistent with the egg-shedding experiment. Samples were held at 4ºC when not being processed. Samples were processed in a manner to obtain a large number of A suum eggs, not for fecal quantification. Processing and sample embryonation was based on several reported methodologies34,70,79-81 and equipment availability. Ascaris suum eggs were isolated using a method similar to the modified Wisconsin sugar flotation method, but adjusted for a large sample volume as follows. Samples were homogenized by hand within the collection bags, and a 100-gram sub-sample was weighed out and placed in a 1-L plastic container. Feces was then mixed with 200 mL of tap water and homogenized in the container using a kitchen potato masher. The fecal-water homogenate was then strained through a large tea strainer into a second 1-L plastic container to remove large-particle organic material. Up to 200 mL of the strained contents was then poured into 200-mL dilution bottles. The bottles were centrifuged at 145g for 10 minutes in a large bucket centrifuge. The pellet was re-suspended in Sheather’s solution to a volume of 200 mL, which was then centrifuged at 145g for 10 minutes. The suspension was allowed to stand for a minimum of 10 minutes, then the top 10 to 15 mL was poured off into 50-mL conical centrifuge tubes. Tap water was added up to 45 mL and the suspension was homogenized manually by vigorous shaking. The tube was centrifuged at 145g for 10 minutes, then the pellet was re-suspended in 30 mL of 0.1 N H2SO4 and transferred into a 50-mL filtered top culture flask (item #10062-872; VWR Radnor, Pennsylvania). Egg concentration was evaluated by counting eggs in a 10-µL sub-sample at 40× magnification. Samples with > 25 eggs per µL were diluted with 0.1 N H2SO4 to achieve this maximum concentration, as it has been reported that egg density influences development.82 Culture flasks were held at 4ºC until embryonation incubation was initiated for all samples within an experiment.
The embryonation period occurred independently for each experiment, once all samples from all collection days were processed. When all samples within an experiment had been processed, culture flasks were simultaneously incubated at room temperature (approximately 23ºC to 25ºC) in the dark for 60 days to ensure complete larval development.79 Flasks were agitated by hand three to four times per week for aeration. At the end of 60 days, flasks were held at 4ºC until embryonation rates could be evaluated.
To calculate embryonation rates, culture flasks were shaken and a 10-mL subsample was poured into a 15-mL conical centrifuge tube and centrifuged at 145g for 10 minutes. The pellet was re-suspended in 5 mL of Sheather’s solution. A volume of Sheather’s solution was added in order to create a meniscus at the top of each tube. A 22 × 22 mm glass coverslip was placed on top of each tube for a minimum of 10 minutes. Coverslips were removed and placed on a glass microscope slide then examined under 100× magnification for A suum eggs presence and embryonation stage. The first 100 A suum eggs observed were evaluated and determined to be either fully embryonated (larvae visualized) or unembryonated, including any stage of development from one-cell to pre-larval stages.83 If fewer than 100 eggs were visualized on a coverslip, additional 10-mL sub-samples were processed until 100 total eggs were counted. Failure to count 100 total eggs from a culture flask resulted in exclusion of that sample from the experiment. Percent of embryonation was recorded as the number of eggs containing fully embryonated larvae out of 100 eggs.
Statistical analysis
All statistical analyses were performed using Enterprise Guide 5.1 software (SAS Institute, Inc, Cary, North Carolina). In each case, sow was considered the experimental unit. The dependent variable in each shedding experiment was the duration of fecal A suum egg shedding, measured as the time to first negative fecal exam for experimental sows. Survival analysis with log-rank test (PROC LIFETEST) was used and the model included treatment group, farm (in the case of experiments), parity, and treatment-farm interaction fixed effects, and the random effect of sow within farm by treatment group. Through backward elimination, effects determined non-significant on the basis of analysis were dropped from further analysis. Comparisons between treatment groups were made using Kaplan-Meier methods as an estimation for survival function from life-time data. Mean survival times (± standard error) were estimated for each treatment. Additionally, the percentage of animals that produced a negative fecal exam by the end of the study was evaluated using chi-square and Fisher’s exact test methods. A calculated mean environmental burden value for each treatment group was evaluated using analysis of variance (ANOVA) methods to evaluate treatment effects, and treatment means were separated using Tukey’s studentized range test. The model implemented included treatment group, farm, parity, and treatment-farm interaction fixed effects. The environmental burden calculation was an attempt to compare observed eggs per gram of feces (EPG) excreted (EPGobs) for the duration of the study period compared to an expected eggs excreted (EPGexp) for each sow and between treatments as a measure of the potential environmental contamination provided by each group. The EPGexp value was that individual’s initial (day 0) EPG multiplied by the number of study days. The EPGobs value was calculated as the cumulative sum of EPG on one sampling day (Di) times the number of days until just prior to the next sampling day (Di+x) through the duration of the study [∑(EPGDi × (Di+X – Di))]. For example, in an animal with sample day EPG values of 100, 80, and 20 on days 0, 4, and 8 respectively, the EPGexp for this animal is 900 [100 EPGDay0 × 9 total days], the EPGobs is 740 [(100 EPGDay0 × 4 days)+(80 EPGDay4 × 4 days) + (20 EPGDay8 × 1 day)], and the environmental burden is 0.822 (740 EPGobs per 900 EPGexp). This environmental burden estimate indicates that the individual shed 82.2% of the eggs over the study duration as was anticipated for that individual.
For embryonation rates, ANOVA methods were used to evaluate treatment effects. When ANOVA effects were significant, treatment means were separated using Tukey’s studentized range test. Analyses were conducted separately for each embryonation experiment because of differences in the sampling protocol followed. In each case, sow was considered the experimental unit. The dependent variable in each study was the percent embryonated A suum eggs (mean embryonation rate), measured as the number of eggs with visible larvae from the 100 eggs evaluated. Independent variables considered were treatment, sampling day, and a treatment-sampling day interaction.
Results
The number of sows in each experiment and between treatment groups varied considerably due to the method of enrollment described, in which a rapid and efficient survey of a large number of sows (eg, 100 to 250) was conducted.
Egg-shedding experiments
In shedding Experiment 1, there were no recorded samples above 500 eggs per gram. In Experiment 2, two Treatment 1 samples and three Treatment 2 samples were above 500 eggs per gram at day 0. In Experiment 3, a single Control sample at days 8 and 10 and one Treatment 2 sample at day 0 exceeded 500 eggs per gram. In Experiment 4, eleven control samples exceeded 500 eggs per gram, with 10 of the 11 coming between days 8 and 22 of sampling, while 10 Treatment 1 and six Treatment 2 samples at day 0 exceeded 500 eggs per gram. One Treatment 1 day 8 sample exceeded 500 eggs per gram in Experiment 4. In Experiment 5, one Treatment 2 and one Treatment 3 day 0 sample each exceeded 500 eggs per gram, as did one Control sample on days 8, 10, and 21.
In the survival analyses, there were significant farm (experiment) effects (P < .001) and evidence of a trend in farm-treatment interaction (P = .054) in the full model; therefore, data was analyzed and reported independently by experiment in a reduced model. There was no parity effect, and parity was excluded from the reduced models. In each experiment, there was a high censoring rate in the control groups (range 70.0% to 100.0%), as many subjects remained positive throughout the period of testing. Kaplan-Meier survival analysis revealed a significant difference in time-to-negative egg shedding between controls and each treatment group. In all shedding experiments, Treatment 1, Treatment 2, and Treatment 3 did not differ in time-to-negative shedding. Complete mean time-to-negative, standard error, and range for each experiment are shown in Table 1. A graph of the survival analysis has been included (Figure 1) for the combined data from experiments 1 through 5. Note that due to the different durations of experiments 1 through 5, the control group contains multiple censored data points, while the largest number of sows censored in any treatment group by experiment was one. Therefore, this graph considerably underestimates the impact of fenbendazole treatments and is a conservative assessment.
Figure 1: Survival analysis: Kaplan-Meier survival curves for time-to-negative fecal Ascaris suum egg shedding from five experiments (data combined) across three commercial sow farms comparing three fenbendazole dosages and treatment periods to treat A suum in naturally infected gestating sows. Duration of treatment differed among the five experiments; therefore, in-phase censoring of control sows is over-represented. Study described in Table 1.
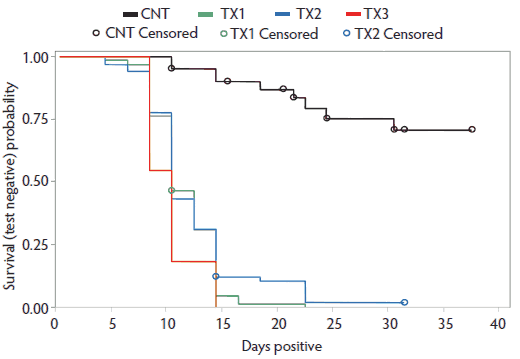
When all shedding experiments were combined, only nine of 40 control animals (22.5%) were negative for A suum eggs throughout the respective study periods. Among the treatment groups, 66 of 67 (98.5%) for Treatment 1, 65 of 67 (97.0%) for Treatment 2, and all of 11 (100.0%) for Treatment 3 were negative for A suum eggs at completion of their respective experiments. The percent negative at end of study differences between control and each treatment was significant by Fisher’s exact test (P < .001). There was no difference in percent negative at end of study among the fenbendazole treatments in any of the five experiments. The results for each experiment are shown in Table 1.
For environmental burden analysis, a significant effect of treatment (P < .001) and farm (P < .001) and a trend in the treatment-farm interaction (P = .054) were observed; therefore, data was analyzed and reported by experiment. The environmental burden analysis demonstrated a significant difference (P < .05) between control and all fenbendazole treatments in each experiment (Tukey’s studentized range test). There were no differences among treatments in any of the five experiments. The environmental burden estimates for controls in experiments 1, 3, and 5 were greater than 100%, representing increasing eggs per gram counts on subsequent samplings from individual control sows. Average environmental burdens for each experiment are listed in Table 1.
It is valuable to note that during collection, most notably on days 6 and 8 post treatment, many of the treated animals were actively expelling adult ascarids (visually observed or manually extracted during rectal sample collection), presumably due to treatment effects of fenbendazole.
Embryonation experiments
In experiments 2 and 3, the independent variables of treatment, sampling day, and a treatment-sampling day interaction were significant sources of variation; therefore, analyses were conducted for each sampling day within each experiment. In all experiments, embryonation rates of controls ranged from 90.3% to 99.3%. Ovicidal activity within treatments was realized on day 4 post treatment, with significant embryonation rate reduction in Treatment 3 (P < .01) and numerical differences in Treatment 1 and Treatment 2 in Experiment 3. After day 4 post treatment (day 6 in Experiment 3 and day 8 in experiments 1 and 2), all treatments were significantly different (P < .001) from controls for each experiment. Complete embryonation rates and standard deviations for all embryonation experiments are shown in Table 2.
The only differences in embryonation rate between fenbendazole dosages were observed on day 4 post treatment in Experiment 3. A numerical difference in embryonation rate was observed in treatments 1 and 2, although it was not statistically significant when compared to controls. By day 6, a difference was no longer observed among treatments and all were different from controls.
It is important to note that during microscopic observation, development was often atypical or arrested in unembryonated eggs in samples from treated sows. Eggs commonly had unequal cleavages, satellite and clustering of blastomeres, smaller, more circular shells, a granular and crystalline appearance to the yolk, lack of any apparent development, and abnormal shapes (Figure 2).
Figure 2: Micrographs of embryonated and unembryonated Ascaris suum eggs after expulsion in three experiments comparing the effects of three fenbendazole treatment regimens on ovicidal activity of A suum eggs shed from naturally infected gestating sows. Panel A: Normally developed larvae, after embryonation, from ova isolated from sows on day 0, prior to treatment with fenbendazole (99% embryonation rate); Panel B: Mix of abnormally developed and arrested eggs after embryonation; unequal cell division (asterisk), satellite blastomeres (arrowheads), and crystalline-like yolk (arrows). Samples collected day 8 after treatment with 545.5 mg fenbendazole on a single day (21% embryonation rate). Studies described in Table 1 and Table 2.
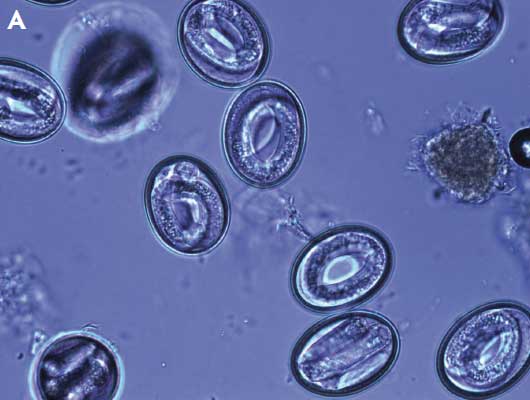
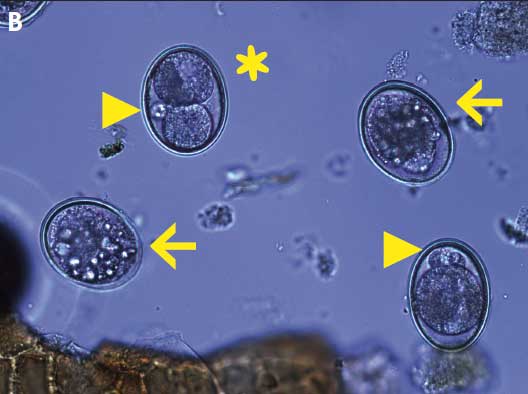
Discussion
Statistical comparison of sow eggs per gram was not conducted due to the inherent high variability of egg counts when assessing A suum infection. Fecal egg counts are highly variable over time within the same subject, as well as within the same fecal passing.44,84 Rather, consistent with the main objectives of the experiments, we assessed the time to cessation of shedding and percent negative sows at the end of respective study periods. In addition, we utilized a calculated environmental burden, which provides a better description of egg shedding, as it uses fecal eggs per gram counts over several time periods to estimate total fecal egg shedding during a period of time. Others have utilized similar calculated estimates of egg contamination. Bernardo et al6,85 used the average eggs per gram counts to calculate a “lifetime burden” in market pigs and modeled the growth impacts of ascariasis. Mejer and Roepstorff86 calculated a “relative contamination index” from fecal eggs per gram of Trichuris suis and Oesophagostomum dentatum in pastured pigs in an attempt to compare contamination rates between experimental paddocks.
The environmental burden calculation was utilized to demonstrate the impact of fenbendazole on reduction of total A suum eggs excreted into the environment, which is an important epidemiological aspect of A suum control. As can be noted by the higher environmental burden values of controls in shedding experiments 1, 3, and 5, non-treated animals may perpetuate or increase fecal egg shedding, and thus contamination of the environment persists over time.
The environmental burden values in shedding Experiment 2 differed statistically between control (79.9%) and treatments 1 (60.9%) and 2 (60.8%), but with less numerical difference between the treatments than in the other shedding experiments. This is likely due to several factors, including the shorter duration of the study (14 days), the number of sampling days (4), and the bias of using a “cutoff” in initial fecal eggs per gram values.
It is important to note that in some fecal samples, eggs per gram was in excess of the 500 eggs per gram cutoff used, and thus introduced bias into the environmental burden calculation. Overall, the use of an eggs per gram cutoff in the shedding experiments resulted in an underestimation of the impact of reduced fecal shedding.
All but one of the above observations (Experiment 4, Treatment 1, day 8 sample) would have resulted in underestimation of the actual environmental burden calculation, since sample cutoff at day 0 would have resulted in a lower expected environmental burden value, and control samples cutoff post treatment would have underestimated the lower environmental burden compared to treatment groups. This could explain the lower numerical differences and reduction in control environmental burden values observed in shedding experiments 2 and 4 compared to experiments 1, 3, and 5.
False-positive results due to low fecal eggs per gram counts or coprophagia,87 when using a highly sensitive test such as the modified Wisconsin sugar flotation technique, are potential sources of misclassification bias. It should be noted that the flotation method used was selected specifically because of its greater sensitivity (approximately 1 egg per gram) relative to other detection methodologies78,88,89 and its common use in North American swine parasitology. In contrast, many European studies utilize a modified McMaster’s technique with a low limit of detection (20 eggs per gram) and recommend a cutoff (200 eggs per gram) to minimize the false-positive effect.44,87 For example, if a cutoff of 200 eggs per gram had been used in the present studies, approximately 50.3% of included sows (93 of 185 sows) would have been excluded for false-positive counts at the time of sow enrollment. Since necropsy and daily adult worm recovery from enrolled sows was not practical or performed, it is impossible to classify each positive sample result as true-positive or false-positive; however, a few observations support using these data collectively as valid results. First, all sows were housed individually in partially slatted gestation stalls and had limited access to feces. Second, many enrolled females from the treatment groups passed adult ascarids, which is consistent with observations by Boes et al.34 Unfortunately this was not recorded. Third, the apparent parasite burden (measured as eggs per gram) for sows in published papers from Europe is much greater when compared to observed eggs per gram values from the present studies and experience in other North American sow herds by the authors36,37 (GHM, personal observation, May 2014). The reason for this discrepancy between reports and geography are not fully understood, but may be related to diagnostic methodology (the McMaster’s technique uses a multiplicative calculation and tends to overestimate eggs at the higher concentrations)88 or inherent management differences (provision of bedding), medication restrictions (anthelmintic use), farm types (multiple ages), and facility designs (group gestation), which result in an overall heavier environmental parasite burden. Another possibility is an inherent geographical variation in egg shedding by adult female parasites, such as has been documented in A lumbricoides.90 Lastly, a decrease in eggs per gram over time was consistently observed throughout each treatment group within each experiment in the present study, while the majority of control animals continued to shed eggs throughout the sampling period, indicating an effect of the treatment on fecal egg shedding.
Future work could minimize the impact of false-negatives by repeated sampling and evaluation of fecal eggs per gram beyond the initial negative test. False-negatives would affect the survival analysis by overestimating the impact of the treatment on treated sows relative to the control sows. Both types of misclassification, false-positive and false-negative, may occur in subjects of both the treatment and control groups.
Some eggs collected for the embryonation experiments might have been associated with coprophagia.87 While this type of false-positive diagnosis is important in evaluating infection prevalence and response to treatment, it may not be a significant issue in embryonation studies, since eggs resulting from coprophagia still contribute to environmental contamination and are susceptible to the ovicidal effects of fenbendazole. Benzimidazoles have significant ovicidal activity in vitro, regardless of whether the eggs are extracted from adult worm uteri or collected from feces.66,67,70,91 Fenbendazole is maintained at a greater in vitro concentration in the gut lumen during the treatment period due to low bioavailability (27.1%);92 44% to 50%60 of the drug remains unchanged and is excreted in the feces, and therefore may have ovicidal activity in eggs in the lumen during treatment periods, although this has not been demonstrated directly. Benzimidazole’s ovicidal activity can affect the embryo even after development is initiated (eg, eggs ingested from the environment after a period of development). Eggs that developed for 9 to 10 days and then were exposed to thiabendazole in vitro ceased further development.67
In the present study, fenbendazole began to have ovicidal effects as early as day 4 post treatment at the single-day 1636.5-mg dosage (Treatment 3), and across all treatments by day 6 through 8 post treatment. The lack of a statistical difference in embryonation rate observed in treatments 1 and 2 on day 4 may have been a result of inadequate sample size to show a difference, or may represent an early dose effect. Effect on embryonation beyond day 8 was not evaluated. Other investigators have reported rapid ovicidal effects of other helminth eggs after treatment with various benzimidazoles in sheep (8 hours)91,93 and humans (24 to 72 hours).70,81,94-97 The more rapid ovicidal activity in these studies, when compared to the present data, may be related to differences in parasite susceptibility, host-parasite interactions, or pharmacokinetics and pharmacodynamics of the anthelmintics for the different host species.
The observations made of atypical egg development, such as unequal cleavages, satellite blastomeres, and crystalline appearance of the yolk, were in agreement with others.67,97,98 Microtubules are important for cell structure, proper cleavage, chromosome movement during cell division, and thus embryogenesis.71 Affected eggs often have irregular shapes, atypical blastomeres, and unequal divisions, which result in irreversible arrested development.34,60,67,98
It is important to note that A suum eggs shed from hosts (ie, breeding sows) are not directly infective to the offspring, an important epidemiological aspect often misunderstood by producers and veterinarians.45,58,79 Freshly shed A suum eggs require a developmental period in the environment outside the host, consisting of two moltings to an infective L3 larvae.99-101 This development usually takes 1 to 3 months or longer, depending on temperature, humidity, and seasonal climate.45,79,101-105 It is this external non-infectious developmental period that allows for basic sanitary and husbandry control measures to be effective if applied correctly. In modern swine farms, with early weaning at 3 weeks of age or less, A suum transmission directly from dam to offspring is unlikely, due to inadequate time for embryo development to an infectious L3. Multivariable risk factor analysis in 413 Scandinavian herds demonstrated that wean age was a significant factor in growing pigs having ascariasis.106 Farms that weaned pigs at greater than 6 weeks of age were twice as likely to have finishers positive for A suum, when compared to farms that weaned between 3 and 5 weeks of age, suggesting that the additional time exposed to farrowing facilities allowed for development of A suum eggs to an infectious stage. In other studies, age-segregated pork production that results in moving pigs to facilities located at a distance from each other reduced the correlation between sow herd A suum status and the A suum status of grow-finish pigs originating from the same sow herd107,108 Transmission to offspring is most likely from older on-site animals (finishing, gilts) or contaminated facilities (finisher barns, gilt development units).109 Indirect transmission is still a concern where piglets may be exposed to infectious eggs remaining in the farrowing environment from previous groups,109,110 poorly sanitized farrowing facilities that allow maintenance of “hot spots” as described by Nilsson,111 or by mechanical transmission from other farm areas that are contaminated (eg, breeding, gestation, gilt development, finishers), by the sow (eg, fecal matter on skin, feet), stockpersons (hands, clothing, boots), and fomites.
Connan103 evaluated development of A suum eggs placed in a commercial swine farm (in England) in order to simulate normal environmental conditions and seasonal influences. Unembryonated eggs placed in June and July became infectious in August and early September, while eggs placed in August and September underwent partial development, then experienced arrested development when conditions were unfavorable (ie, winter), and resumed development the following spring, although with reduced percent embryonation.103 Eggs placed from September through May developed synchronously in the subsequent July. This seasonal development may be recognized as seasonal variations in liver condemnation rates at slaughter plants, with the greatest prevalences seen July through December, when growing pigs exposed to infectious eggs are marketed.112,113 Seasonal development is seen in pasture-raised pigs, where a “spring rise” and increasing prevalence is observed when pastures are infected the prior fall.49,86,114 On the other hand, it is expected that environmentally controlled facilities, such as farrowing rooms and stalls with supplemental heat (eg, heat lamps, heat mats, brooder covers), would promote larval development year round.115
The US label for Safe-Guard EZ Scoop states “For individual 400 lb sow feeding: Mix 1 level scoop (1.07 ounces) of Safe-Guard EZ Scoop premix into 4 to 6 lb of an individual 400 lb sow’s daily ration and feed once daily for 3 consecutive days.” However, extra-label treatment of sows with a single-day, single-scoop dose of Safe-Guard EZ Scoop (ie, Treatment 1), equating to a 3 mg per kg dose for a 181.8-kg animal, is commonly used. In addition, farms usually do not weigh individual sows prior to treatment, but rather estimate an average weight for all sows72 (JSP, personal observation, May 2014). Therefore, treatment may be less than indicated by both dose and frequency. The consequence of imprecise dosing and abbreviated treatment regimens on A suum egg shedding has not been thoroughly evaluated. In early studies of fenbendazole in swine, Baeder et al59 used a single oral dose of 5 mg per kg and eliminated 100% of adult A suum from the intestine, and Batte61 demonstrated the efficacy (96.0% adult A suum removed) of 3 mg per kg as a single dose. Extended treatment (3 days or longer) with fenbendazole, even with a lower daily dose, is usually considered more effective than single doses, namely, for treatment of Trichuris suis.61,116 Fenbendazole was used in an off-label manner in this study; however, the authors do not necessarily recommend off-label use under field conditions. It was the authors’ intent to mimic the potential application variation of this product as it might be used in the field by pork producers and veterinarians.
The results of the shedding experiments support using fenbendazole in breeding females prior to farrowing, and indicate that treatment is highly effective and should begin 14 days prior to moving sows into clean farrowing facilities in order to minimize A suum egg shedding into that environment. Treatment of breeding-herd animals after this time, or upon entry into the farrowing facility, will likely result in increasing facility contamination and increasing transmission risk to suckling piglets due to carryover of embryonated eggs from previous farrowing groups.
The embryonation experiments demonstrated that fenbendazole has ovicidal activity at various treatment levels on A suum eggs shed from naturally infected sows, applied in a manner consistent with practical commercial farm methods. Results from these experiments agree with work by others who have evaluated the ovicidal activity of other benzimidazoles on A suum and A lumbricoides or fenbendazole on other parasites.33,66,67,70,91 To the authors’ knowledge, this is the first description of the ovicidal activity of fenbendazole in A suum eggs collected from the feces of naturally infected sows.
In addition to sanitation and anthelmintic treatment to reduce adult worm burden and shedding by hosts, using an effective anthelmintic with ovicidal activity, such as fenbendazole, adds an epidemiological advantage by reducing the effective infectious egg load in the environment. Fenbendazole, with its adulticidal, larvacidal, and ovicidal properties, provides added value when implemented in A suum control programs in an infected population.
Implications
• Under the conditions of this study, fenbendazole, as a feed top-dress at 545.5 mg for 1 day, 545.5 mg daily for 3 consecutive days, or 1636.5 mg for 1 day, is effective for A suum treatment in naturally infected gestating breeding sows.
• Breeding female swine with naturally occurring A suum should be dewormed with fenbendazole at least 14 days prior to entry into clean farrowing facilities to minimize transmission to offspring and reduce facility contamination.
• Under the conditions of this study, fenbendazole, at 545.5 mg for 1 day, 545.5 mg daily for 3 consecutive days, or 1636.5 mg for 1 day, is ovicidal to A suum eggs shed from naturally infected gestating sows, starting as soon as 4 days post treatment and lasting through at least 8 days post treatment.
• Fenbendazole as a treatment for A suum provides an additional epidemiological advantage through reducing effective environmental contamination resulting from its ovicidal properties.
Acknowledgements
This research was supported by funding from Murphy-Brown, LLC. The primary author would like to thank the farm management and staff at the sites in which these studies were conducted, and the numerous veterinary students who assisted with sample collection during the research project time period.
Conflict of interest
None reported.
Disclaimer
Scientific manuscripts published in the Journal of Swine Health and Production are peer reviewed. However, information on medications, feed, and management techniques may be specific to the research or commercial situation presented in the manuscript. It is the responsibility of the reader to use information responsibly and in accordance with the rules and regulations governing research or the practice of veterinary medicine in their country or region.
References
1. Roepstorff A, Nansen P. Epidemiology and control of helminth infections in pigs under intensive and non-intensive production systems. Vet Parasitol. 1994;54:69–85.
2. Greve JH. Internal parasites: Helminths. In: Zimmerman JJ, Karriker LA, Ramirez A, Schwartz KJ, Stevenson GW, eds. Diseases of Swine. 10th ed. Ames, Iowa: Wiley-Blackwell; 2012:908–920.
3. Hale OM, Stewart TB, Marti OG. Influence of an experimental infection of Ascaris suum on performance of pigs. J Anim Sci. 1985;60:220–225.
4. Stewart TB, Hale OM. Losses to internal parasites in swine production. J Anim Sci. 1988;66:1548–1554.
*5. Nilsson O, Martinsson K. The influence of subclinical ascariasis on growing pigs. Proc IPVS. Copenhagen, Denmark. 1980;265.
6. Bernardo TM, Dohoo IR, Donald A, Ogilvie T, Cawthorn R. Ascariasis, respiratory diseases and production indices in selected Prince Edward Island swine herds. Can J Vet Res. 1990;54:267–273.
7. Kipper M, Andretta I, Monteiro SG, Lovatto PA, Lehnen CR. Meta-analysis of the effects of endoparasites on pig performance. Vet Parasitol. 2011;181:316–320.
*8. Hurnik D, Dohoo IR. The impact of roundworms on swine production. Comp Cont Educ Pract Vet. 1995;17:589–593.
*9. Harr BS, Bowman GL, Rajala-Schultz P, Moeller SJ. Liver condemnations as a result of ascarid infection. Proc AASV. Nashville, Tennessee. 2001;65–67.
10. McOrist S, Blunt R, Wiseman J. Ascaris suum parasitism in finisher pigs: welfare and economic impacts and control options. Pig J. 2008;61:74–79.
11. USDA:APHIS:VS. Swine 2006 Part II: Reference of Swine Health and Health Management Practices in the United States, 2006. Fort Collins, Colorado: National Animal Health Monitoring System (NAHMS). 2007;N479.1007. Available at: http://www.aphis.usda.gov/animal_health/nahms/swine/downloads/swine2006/Swine2006_dr_PartII.pdf. Accessed 24 February 2015.
12. Boes J, Kanora A, Havn KT, Christiansen S, Vestergaard-Nielsen K, Jacobs J, Alban L. Effect of Ascaris suum infection on performance of fattening pigs. Vet Parasitol. 2010;172:269–276.
13. Urban JF Jr, Steenhard NR, Solano-Aguilar GI, Dawson HD, Iweala OI, Nagler CR, Noland GS, Kumar N, Anthony RM, Shea-Donohue T, Weinstock J, Gause WC. Infection with parasitic nematodes confounds vaccination efficacy. Vet Parasitol. 2007;148:14–20.
14. Steenhard NR, Jungersen G, Kokotovic B, Beshah E, Dawson HD, Urban JF Jr, Roepstorff A, Thamsborg SM. Ascaris suum infection negatively affects the response to a Mycoplasma hyopneumoniae vaccination and subsequent challenge infection in pigs. Vaccine. 2009;27:5161–5169.
15. Shope RE. The swine lungworm as a reservoir and intermediate host for hog cholera virus. I. The provocation of masked hog cholera virus in lungworm-infested swine by ascariasis larvae. J Exp Med. 1958;107:609–622.
16. Underdahl NR, Kelley GW. The enhancement of virus pneumonia of pigs by the migration of Ascaris suum larvae. JAVMA. 1957;4:173–176.
17. Nayak DP, Kelley GW. Effect of ascaris migration and influenza infection in mice. J Parasitol. 1965;51:297–298.
*18. Matusyavichus AP, Danilyavichus EA, Shpakauskas VI. Disease of pigs caused by association of helminths and erysipelas [abstract]. Pig News Inform. 1985;6:333.
19. Curtis SE, Tisch DA, Todd KS, Simon J. Pulmonary bacterial deposition and clearance during ascarid larval migration in weanling pigs. Am J Vet Res. 1987;51:515–527.
20. Adedeji SO, Ogunba EO, Dipeolu OO. Synergistic effect of migrating Ascaris larvae and Escherichia coli in piglets. J Helminthol. 1989;63:19–24.
21. Tjørnehøj K, Eriksen L, Aalbæk B, Nansen P. Interaction between Ascaris suum and Pasteurella multocida in the lungs of mice. Parasitol Res. 1992;78:525–538.
*22. Vyt P, De Bie S, Kanora A. Correlation between white liver spots and pneumonia: a slaughterhouse survey. Proc IPVS. Hamburg, Germany. 2004;2:582.
*23. Engle MJ, Bush EJ. Risk factors associated with PMWS in US swine herds. Proc IPVS. Copenhagen, Denmark. 2006;1:172.
*24. Jourquin J, Jacobs J, van Gelderen R. Impact of migrating Ascaris suum larvae on the respiratory disease complex. Proc IPVS. Durban, South Africa. 2008;1:197
*25.Perry A, Stokappe J. Ascarid larval pneumonia in pigs [Cross-Canada Disease Report]. Can Vet J. 1993;34:58.
*26. Gjestvang M, Lium B, Gamlem H, Gjerde B. Acute respiratory distress and death caused by migrating larva of Ascaris suum in finishing pigs. Proc IPVS. Hamburg, Germany. 2004;2:841.
*27. Trujano M, López J. Respiratory problems related to Ascaris suum in pigs. A case report. Proc IPVS. Cancun, Mexico. 2014;2:124.
28. Knecht D, Janakowska A, Zaleśny G. The impact of gastrointestinal parasites infection on slaughter efficiency in pigs. Vet Parasitol. 2012;184:291–297.
29. Knecht D, Popiołek M, Zaleśny G. Does meatiness of pigs depend on the level of gastro-intestinal parasites infection? Prev Vet Med. 2011;99:234–239.
30. Bendall RP, Barlow M, Betson M, Stothard JR, Nejsum P. Zoonotic ascariasis, United Kingdom. Emerg Infect Dis. 2011;17:1964–1965.
31. Dold C, Holland CV. Ascaris and ascariasis. Microb Infect. 2011;13:632–637.
32. Nejsum P, Beton M, Bendall RP, Thamsborg SM, Stothard JR. Assessing the zoonotic potential of Ascaris suum and Trichuris suis: looking to the future from an analysis of the past. J Helminthol. 2012;86:148–155.
33. Zhou C, Li M, Yuan K, Deng S, Peng W. Pig Ascaris: an important source of human ascariasis in China. Inf Genet Evol. 2012;12:1172–1177.
34. Boes J, Eriksen L, Nansen P. Embryonation and infectivity of Ascaris suum eggs isolated from worms expelled by pigs treated with albendazole, pyrantel pamoate, ivermectin or piperazine dihydrochloride. Vet Parasitol. 1998;75:181–190.
35. Myers GH. Strategies to control intestinal parasites in cattle and swine. J Anim Sci. 1988;66:1555–1564.
*36. Johnson R, Myers G. The three M’s of internal parasite control. Proc AASV. Orlando, Florida. 2003:203–204.
*37. Pittman JS, Shepherd G, Thacker BJ, Myers GH, Francisco CJ. Prevalence of internal parasites in a production system: Part I – Sows. Proc IPVS. Vancouver, Canada. 2010;806.
*38. Pittman JS, Shepherd G, Thacker BJ, Myers GH, Francisco CJ. Prevalence of internal parasites in a production system: Part II – Finishing pigs. Proc IPVS. Vancouver, Canada. 2010;807.
*39. Duff J, Myers GH, Thacker B. System wide survey of Ascaris suum eggs in sows housed in penned gestation facilities. Proc IPVS. Cancun, Mexico. 2014;1:229.
*40. Kelley GW, Smith LJ. The daily egg production of Ascaris suum and the inability of low levels of aureomycin to affect egg production and embryonation [research note]. J Parasitol. 1956;42:587.
41. Olsen LS, Kelley GW, Sen HG. Longevity and egg-production of Ascaris suum. Trans Amer Microscop Soc. 1958;77:380–383.
42. Wharton DA. Ascaris sp: Water loss during desiccation of embryonating eggs. Exp Parasitol. 1979;48:398–406.
43. Gaasenbeek CPH, Borgsteede FHM. Studies on the survival of Ascaris suum eggs under laboratory and simulated field conditions. Vet Parasitol. 1998;75:227–234.
44. Roepstorff A, Nansen P. Epidemiology, diagnosis and control of helminth parasites of swine. FAO Animal Health Manual. Rome, Italy: Food and Agriculture Organization of the United Nations. 1998;161 pages.
45. Roepstorff A. Ascaris suum in pigs: population biology and epidemiology. PhD thesis. The Royal Veterinary and Agricultural University, Copenhagen, Denmark. 2003.
46. Dangolla A, Willeberg P, Bjørn H, Roepstorff A. Associations of Ascaris suum and Oesophagostomum spp. infections of sows with management factors in 83 Danish herds. Prev Vet Med. 1996;27:197–209.
47. Haugegaard J. Prevalence of nematodes in Danish industrialized sow farms with loose housed sows in dynamic groups. Vet Parasitol. 2010;168:156–159.
*48. Sundrum A, Werner C. Endoparasite infections and hygiene management in organic fattening herds. Proc IPVS. Vancouver, Canada. 2010;809.
49. Roepstorff A, Mejer H, Nejsum P, Thamsborg SM. Helminth parasites in pigs: new challenges in pig production and current research highlights. Vet Parasitol. 2011;180:72–81.
*50. Eriksen L, Roepstorff A, Nansen P. Prevalence and control of helminth infections in pigs under different management systems. Proc IPVS. Bologna, Italy. 1996;350.
51. Cartensen L, Vaarst M, Roepstorff A. Helminth infections in Danish organic swine herds. Vet Parasitol. 2002;106:253–264.
52. Eijck IAJM, Borgsteede FHM. A survey of gastrointestinal pig parasites on free-range, organic and conventional pig farms in the Netherlands. Vet Res Commun. 2005;29:407–414.
53. Yaeger MJ, Karriker LA, Layman L, Halbur PG, Huber GH, van Hulzen K. Survey of disease pressures in twenty-six niche herds in the midwestern United States. J Swine Health Prod. 2009;17:256–263.
54. Woods AL, Tynes VV. Special considerations for show and pet pigs. In: Zimmerman JJ, Karriker LA, Ramirez A, Schwartz KJ, Stevenson GW, eds. Diseases of Swine. 10th ed. Ames, Iowa: Wiley-Blackwell; 2012:179–185.
55. Raffensperger HB, Connelly JW. The swine sanitation system as developed by the bureau of animal industry in McLean County, Ill. USDA Tech Bull. 1927;44:1–19.
*56. Behlow RF, Batte EG. North Carolina Swine Parasite Control Program. North Carolina Agricultural Extension Service. 1978;AG-111.
*57. Biehl LG, Behlow RF, Batte E. Internal Parasites. Pork Industry Handbook. 1987.
*58. Stewart TB, Stromberg BE, Lawhorn DB. Internal Parasites in Swine. Pork Industry Handbook. 1993.
59. Baeder C, Bahr H, Christ O, Duwel D, Kellner HM, Kirsh R, Loewe H, Schultes E, Schutz E, Westen H. Fenbendazole: a new, highly effective anthelmintic. Experientia. 1974;30:753–754.
60. Düwel D. Fenbendazole. II. Biological properties and activity. Pest Sci. 1977;8:550–555.
61. Batte EG. Evaluation of fenbendazole as a swine anthelmintic. Vet Med Small Anim Clin. 1978;73:1183–1186.
62. Corwin RM, Pratt SE, Muser RK. Evaluation of fenbendazole as an extended anthelmintic treatment regimen for swine. JAVMA. 1984;185:58–59.
63. Stewart TB, Marti OG, Hale OM. Efficacy of fenbendazole against five genera of swine parasites. Am J Vet Res. 1981;42:1160–1162.
64. Stewart TB, Binder TD, Southern LL, Simmons LA. Efficacy of fenbendazole against migrating Ascaris suum larvae in pigs. Am J Vet Res. 1984;45:984–986.
65. Stewart TB, Rowell TJ. Susceptibility of fourth-stage Ascaris suum larvae to fenbendazole and to host response in the pig. Am J Vet Res. 1986;47:1671–1673.
*66. Egerton JR. The effects of thiabendazole upon Ascaris and Stephanurus infections [abstract]. J Parasitol. 1961;47(suppl):37.
67. Egerton, JR. The ovicidal and larvicidal effect of thiabendazole on various helminth species. Texas Rep Biol Med. 1969;27(suppl2):561–580.
68. Friedman PA, Platzer EG. Interaction of anthelmintic benzimidazoles with Ascaris suum embryonic tubulin. Biochim Biophys Acta. 1980;630:271–278.
69. Lacey E, Brady RL, Prichard RK, Watson TR. Comparison of inhibition of polymerization of mammalian tubulin and helminth ovicidal activity by benzimidazole carbamates. Vet Parasitol. 1987;23:105–119.
70. Massara CL, Ferreira RS, Guerra HL, Carvalho ODS. In vitro study on thiabendazole action on viability of Ascaris lumbricoides (Lineu, 1758) eggs. Revista da Sociedade Brasileira de Medicina Tropical. 2001;34:319–322.
71. Martin RJ. Modes of action of anthelmintic drugs. Vet J. 1997;154:11–34.
72. Dangolla A, Bjørn H, Willeberg P, Roepstorff A, Nansen P. A questionnaire investigation on factors of importance for the development of anthelmintic resistance of nematodes in sow herds in Denmark. Vet Parasitol. 1996;63:257–271.
73. Boes J, Medley GF, Eriksen L, Roepstorff A, Nansen P. Distribution of Ascaris suum in experimentally and naturally infected pigs and comparison with Ascaris lumbricoides infections in humans. Parasitology. 1998;117:589–596.
74. Marti OG, Hale OM. Parasite transmission in confined hogs. Vet Parasitol. 1986;19:301–314.
75. Roepstorff A, Nilsson O, Oksanen A, Gjerde B, Richter SH, Örtenberg E, Christensson D. Martinsson KB, Bartlett PC, Nansen P, Eriksen L, Helle O, Nikander S, Larsen K. Intestinal parasites in swine in the Nordic countries: prevalence and geographical distribution. Vet Parasitol. 1998;78:305–319.
76. Nosal P, Petryszal A, Nowosad B. Some aspects of nematode infection in pigs from small herds. Pol J Vet Sci. 2008;11:219–223.
77. David ED, Lindquist WD. Determination of the specific gravity of certain helminth eggs using sucrose density gradient centrifugation. J Parasitol. 1982;68:916–919.
*78. Bliss DH, Kvasnicka WG. The fecal examination: a missing link in food animal practice. Comp Educ Pract Vet: Food Anim Med Management. 1997;April:S104–S109.
79. Arene FOI. Ascaris suum: influence of embryonation temperature on the viability of the infective larva. J Therm Biol. 1986;11:9–15.
80. Okasen A, Eriksen L, Roepstorff A, Ilsøe B, Nansen P, Lind P. Embryonation and infectivity of Ascaris suum eggs. A comparison of eggs collected from worm uteri with eggs isolated from pig feces. Acta Vet Scand. 1990;31:393–398.
81. Massara CL, Costa HMA, Souza DWC, Souza MSL, Carvalho ODS. Viability of Ascaris lumbricoides eggs eliminated after anti-helminthic therapy. Mem Inst Oswaldo Cruz. 1991;86:233–237.
82. Eriksen L. Ascaris suum: influence of egg density on in vitro development from embryonated egg to infective stage. Acta Vet Scand. 1990;31:489–491.
83. Cruz LM, Allanson M, Kwa B, Azizan A, Izurieta R. Morphological changes of Ascaris spp. eggs during their development outside the host. J Parasitol. 2012;98:63–68.
84. Bindseil E. Observations on the relationship between Ascaris suum burdens in pigs and faecal egg counts. Acta Path Microbial Scand Sect B. 1974;82:879–884.
85. Bernardo TM, Dohoo IR, Donald A. Effect of ascariasis and respiratory disease on growth rates in swine. Can J Vet Res. 1990;54:278–284.
86. Mejer H, Roepstorff A. Oesophagostomum dentatun and Trichuris suis infections in pigs born and raised on contaminated paddocks. Parasitology. 2006;133:295–304.
87. Boes J, Nansen P, Stephenson LS. False-positive Ascaris suum egg counts in pigs. Int J Parasit. 1997;27:833–838.
88. Egwang TG, Slocombe JOD. Efficiency and sensitivity of techniques for recovering nematode eggs from bovine feces. Can J Comp Med. 1981;45:243–248.
89. Dryden MW, Payne PA, Ridley R, Smith V. Comparison of common fecal flotation techniques for the recovery of parasite eggs and oocysts. Vet Therapeutics. 2005;6:15–28.
90. Hall A, Holland C. Geographical variation in Ascaris lumbricoides fecundity and its implications for helminth control. Parasit Today. 2000;16:540–544.
91. Kirsch R. In vitro and in vivo studies on the ovicidal activity of fenbendazole. Res Vet Sci. 1978;25:263–265.
92. Petersen MB, Friis C. Pharmacokinetics of fenbendazole following intravenous and oral administration to pigs. Amer J Vet Res. 2000;61:573–576.
93. Southcott WH. Ovicidal effect of thiabendazole and its activity against immature helminths of sheep. Aust Vet J. 1963;39:452–458.
94. Carvalho OS, Guerra HL, Massara CL. Development of Ascaris lumbricoides eggs from females eliminated after chemotherapy in man. Mem Inst Oswaldo Cruz. 1992;87:49–51.
95. Maisonneuve H, Rossignol JF. Ovicidal effects of albendazole in human ascariasis, ancylostomiasis and trichuriasis. Ann Trop Med Parasitol. 1985;79:79–82.
96. Wagner ED, Chavarria AP. In vivo effects of a new anthelmintic, mebendazole (R-17,635) on the eggs of Trichuris trichiura and hookworm. Am J Trop Med Hyg. 1974;23:151–153.
97. Wagner ED, Chavarria AP. Morphologically altered eggs of Trichuris trichiura following treatment with mebendazole. Am J Trop Med Hyg. 1974;23:154–157.
98. Kirsch R, Schleich H. Morphological changes in trichostrongylid eggs after treatment with fenbendazole. Vet Parasitol. 1982;11:375–380.
*99. Stoll NR. When are ascaris eggs infective? [abstract]. J Parasitol. 1933;20:126.
100. Fagerholm HP, Nanse P, Roepstorff A, Frandsen F, Eriksen L. Differentiation of cuticular structures during the growth of the third-stage larva of Ascaris suum (Nematoda, Ascaridoidea) after emerging from the egg. J Parasitol. 2000;86:421–427.
101. Kirchgäßner M, Schmahl G, Al-Quraishy S, Ghaffar FA, Mehlhorn H. What are the infectious larvae in Ascaris suum and Trichuris muris? Parasitol Res. 2008;103:603–607.
102. Seamster AP. Developmental studies concerning the eggs of Ascaris lumbricoides var. suum. Amer Midland Nat. 1950;43:450–470.
103. Connan RM. Ascariasis: the development of eggs of Ascaris suum under the conditions prevailing in a pig house. Vet Rec. 1977;100:421–422.
104. Geenen PL, Bresciani J, Boes J, Pedersen A, Eriksen L, Fagerholm HP, Nansen P. The morphogenesis of Ascaris suum to the infective third-stage larvae within the egg. J Parasitol. 1999:85:616–622.
105. Kim MK, Pyo KH, Hwang YS, Park KH, Hwang IG, Chai JY, Shin EH. Effect of temperature on embryonation of Ascaris suum eggs in an environmental chamber. Korean J Parasitol. 2012;50:239–242.
106. Roepstorff A, Nilsson O, O’Callaghan CJ, Oksanen A, Gjerde B, Richter SH, Ortenberg EÖ, Christensson D, Nansen P, Eriksen L, Medley GF. Intestinal parasites in swine in the Nordic countries: multilevel modeling of Ascaris suum infections in relation to production factors. Parasitology. 1999;119:521–534.
107. Roepstorff A. Transmission of intestinal helminths in Danish sow herds. Vet Parasitol. 1991;39:149–160.
*108. Homgren N, Nilsson O. The influence of age segregated production on the occurrence of white spots in the liver of slaughter pigs. Proc IPVS. Birmingham, England. 1998:111.
*109. Thomas RJ, Ferber M. The epidemiology of helminthiasis in pigs. Proc Pig Vet Soc. Solihull, England. 1983;555.
110. Roepstorff A. Helminth surveillance as a prerequisite for anthelmintic treatment in intensive sow herds. Vet Parasitol. 1997;73:139–151.
111. Nilsson O. Ascariasis in the pig. An epizootiological and clinical study. Acta Vet Scand. 1982;79(suppl):1–108.
112. Goodall EA, McLoughlin EM, Menzies FD, McIlroy SG. Time series analysis of the prevalence of Ascaris suum infections in pigs using abattoir condemnation data. Anim Prod. 1991;53:367–372.
113. Menzies FD, Goodall EA, Taylor SM. The epidemiology of Ascaris suum infections in pigs in Northern Ireland, 1969-1991. Br Vet J. 1994;150:165–172.
114. Larsen MN, Roepstorff A. Seasonal variation in development and survival of Ascaris suum and Trichuris suis eggs on pastures. Parasitology. 1999;119:209–220.
*115. Murrell KD. Epidemiology, pathogenesis, and control of major swine helminth parasites. Vet Clin North Am Food Anim Pract. 1986;2:439–454.
116. McKellar QA, Scott EW. The benzimidazole anthelmintic agents – a review. J Vet Pharmacol Therap. 1990;13:223–247.
*Non-refereed references.